A challenge for the biotech community to grow toward the biggest problems.
It’s a great time to be a mouse with cancer. Billions of dollars have been invested in curing you and inventing new technology stacks to cure all your subtypes. In contrast, it’s not a great time to be a whale, or a pine tree, or any biological resident of East Palestine, OH.
Why? Because the better the economic case, the easier it is to mobilize human capital. In medicine, this has taken us to a strange place where dozens of companies work to solve the same, “commercially-viable” diseases[1]. Too many amazing people work on problems with high professional gravity, rather than truly impactful solutions. It’s great that many deadly diseases are now curable, and that a vaccine for the novel coronavirus was prototyped within days and distributed in months. But human resources are still not allocated toward the most important problems in biotech. This paradox is what prompted Peter Thiel to lament, one decade ago, “we were promised flying cars, instead we got 140 characters.”
Similarly, in bioengineering, we were promised solarpunk[2], but we got antibody-drug conjugates. We wanted fuels grown from algae and instead we barely get pharmaceuticals grown from algae while 98% of our chemical feedstocks still come from oil. And we wanted cell-free manufacturing, but we’re still stuck at laundry detergents.
So how do we work on the most important problems[3]? There’s no shortage of government and private capital looking to boost climate tech, and there’s no shortage of talented bioengineers wanting to work on big climate issues, so clearly there is a disconnect somewhere in the middle. We need to increase connectivity between problems and talents in order for biotech to reach its trillion-dollar potential on a timeline that matters for planetary health.
Potential in scale and precision; Challenge to engineer and deploy.
Biotech is ideally suited to solving planetary-scale challenges because of its two extremes: it is atomically precise and infinitely scalable[4]. But there are several reasons that biology is not yet harnessed fully to address climate change.
For one, biology is hard to engineer[5]. It takes many shots on goal to accomplish much of anything. And when something does work in the lab – usually after burning through lots of time and money[6]– the deployment journey is so long and capital-intensive that you’d better hope the initial result is tied to a translational path that matters.
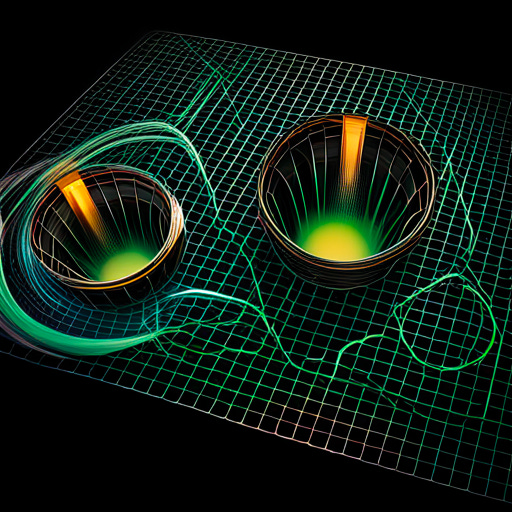
AI may well be an accelerant, but bio science and bio deployment are still difficult in different ways.
Beyond just “Life finds a way” to do what it wants, there are simply not yet many playbooks for successfully deploying biotech commercially. If you work with mammalian cells, you’re probably headed to clinical trials with a biopharma giant. If you’re engineering plants, you probably want to sign a partnership with the Big Four[7] agricultural oligopoly. These trodden paths to impact are interpretable by capital markets, priced accordingly and staffed organically.
The way to ignite more transformative impacts in biotech, then, is to build a broader set of platforms to shepherd early ideas into world-changing impacts. Innovation is needed in how we support early translational science and in how we scale those ideas in absence of established playbooks. Such meta-innovation is essential because the existing drivers for biotech today do not properly incentivize efforts that leverage the strongest features of biology.
Let’s dream about futures that lay on the other side of both engineering and deployment challenges. It’s easy to look backwards and critique, it’s harder to look forwards and lead. Starting from features of atomic precision and infinite scalability, I’ll briefly skim over a few areas that are under-appreciated relative to impact. We will explore more in the months ahead.
Four important bio-based topics that are hard in both science and deployment
Cell-free manufacturing
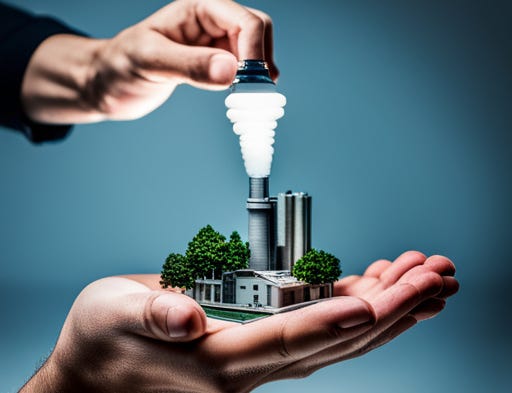
Cell-free manufacturing can use the atomic precision of biology across industrial applications. While there are significant practical challenges, there is no theoretical reason why multi-step chemical processes couldn’t be done at scale by self-assembled molecular conveyor belts. Let’s tangibly imagine modular solutions of cell-free manufacturing to existing industrial problems: could we one day shrink those enormous factories into sets of programmable flow cells that we assemble like LEGOs? One very likely outcome is that proteins (which alone don’t scale that well due to lifespan) will need to be combined with synthetic approaches (see: Cascade, Caravel, Saipem, Solugen and Plexymer), unlocking chemical frontiers beyond today’s commodities. Just as an airplane doesn’t have feathers, it’s very possible that the way we deploy solutions from nature will look very different from the original context.
Open deployment of biotechnology
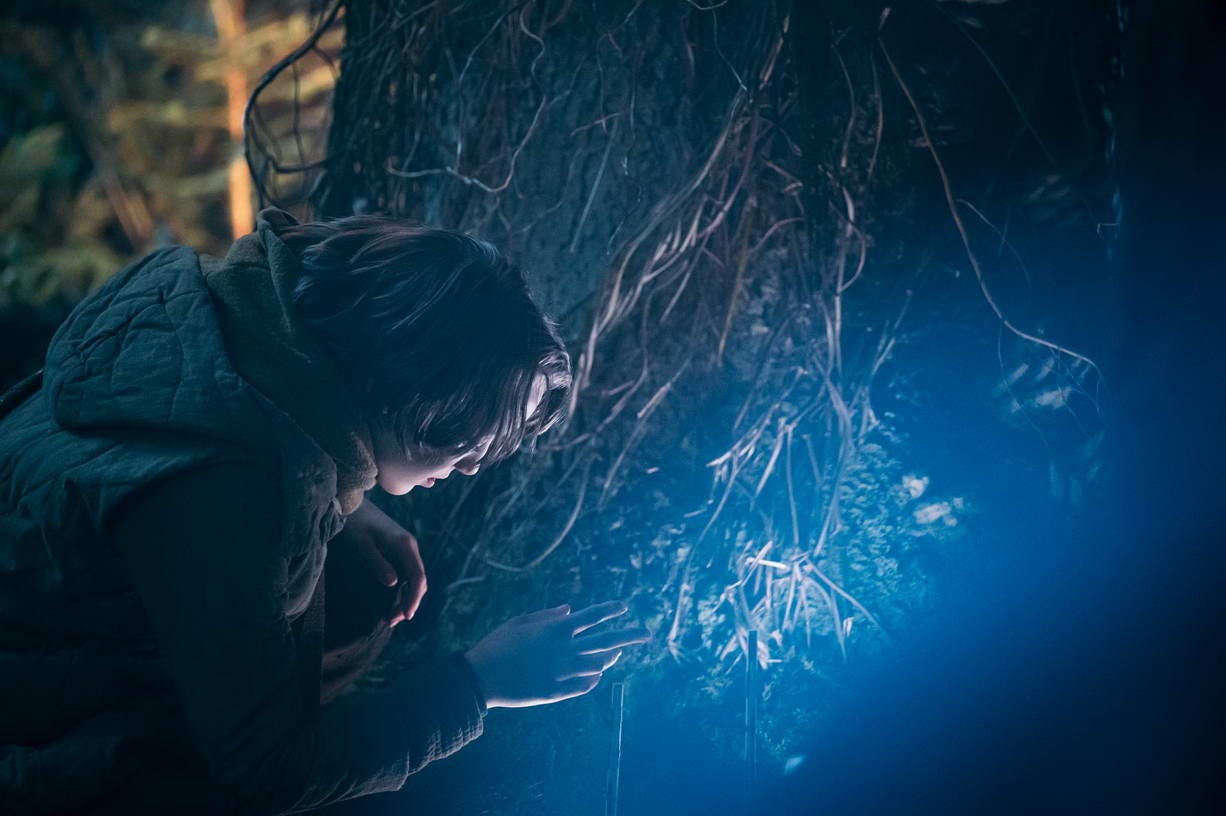
Precision tools could harness the self-scaling aspects of biology safely. Very few labs seriously consider experiments of open deployment systems of genetically engineered organisms. This is understandable: An experiment gone terribly wrong could be catastrophic and so the topic is taboo. Is this fear holding us back? What if there was such a thing as public roadmaps for open deployments that weighed the highest impact goals against risk, all framed as a collective engineering challenge? It is important to build at the frontiers of “Precision ecological medicine,” as default paths are leading to ecosystem collapse, and some geoengineering plans like monocrop trees for carbon capture are flawed yet need more than reductive critique. The future bioengineer’s toolkit must harness biology’s magnificent ability to self-organize from the nanometer to the kilometer.
Pollution-caused diseases
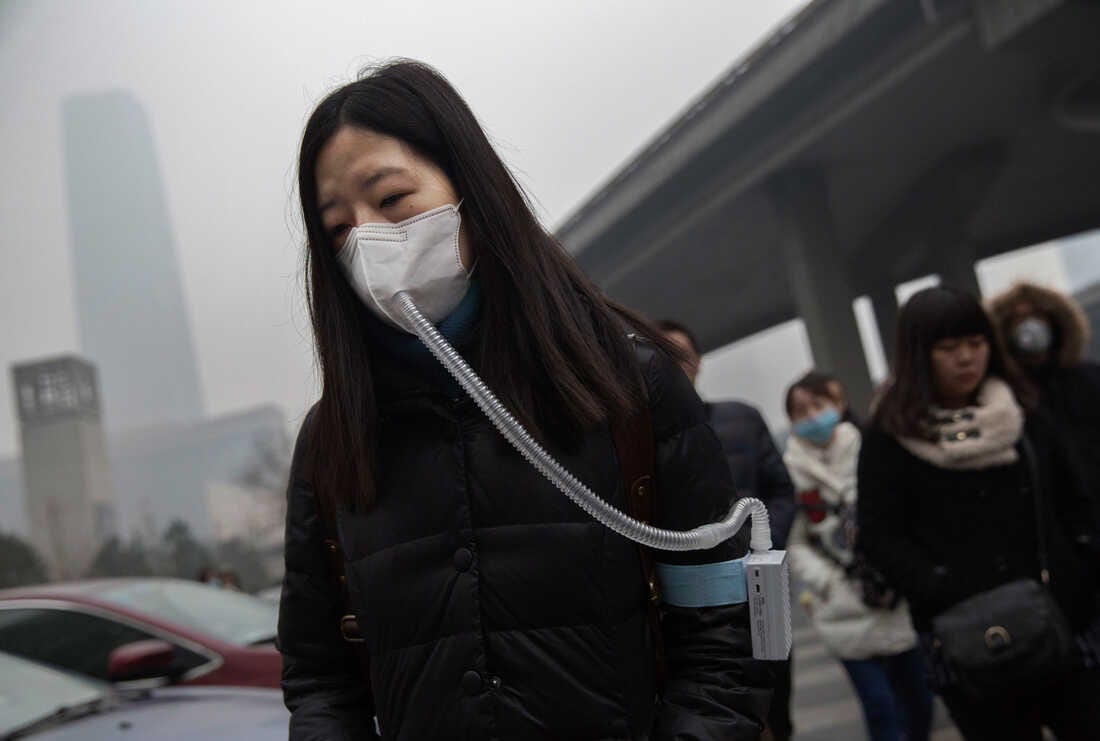
Pathologies caused by environmental issues might be the link between human health and planetary health. It’s easy to ignore melting glaciers or dying species far away, but the human health effects of industrial byproducts are now as proximate as a coal miner’s canary. Cancer rates are increasing rapidly, especially in young people. So too are the rates of autoimmune diseases, infertility and autism disorders. After the best genetic studies have been done on autism, the only conclusion is that genetics alone are little explanation. Then there is the insect collapse and hermaphroditic polar bears. These pan-species disasters indicate some external factors in the environment, yet systematic monitoring is hard: tiny, mostly unregistered molecules interacting in unknown combinations have unknown biological effects in unknown combinations in unmeasurable situations. But we cannot underestimate the power of an activated, well-resourced community effort taking a data-first, molecules-up viewpoint: if we understand the basis what is currently called “sporadic” diseases, we can start to better price the cost of pollution and incentivize cleaner technology.
Microbe and metal interfaces
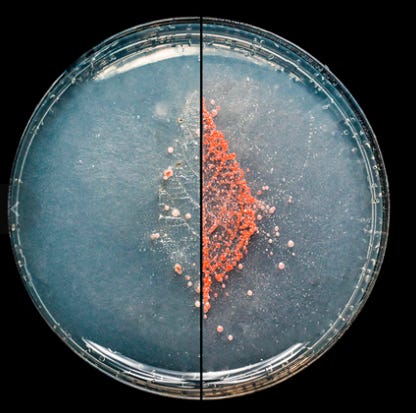
Applied Geobiology becomes the bedrock of a new bioengineering boom era. Organisms isolate specific metal ions and dissolve/construct minerals with high efficiency across the planet. This means geobiology is foundational to our future efforts in mining metals, capturing carbon, and harnessing energy. Despite this potential, geobiology has been historically neglected, or at best, its efforts siloed inside industrial practices: In contrast to medical biotech, geobiologists do not have a dedicated suite of tools for technology development, communication, and data centralization. Geobiology has no Cell Atlases, “Kendall Squares” or “Silicon Valleys.” It would be complementary to government funding efforts to grow connective tissue across siloed projects and lay the foundation for an organically growing public/private community.
Community as a bottleneck
ClimateTech is unique because it is a theme of technology growing under external time pressure. It is a novel challenge for humanity to suddenly deploy a trillion dollars in the face of both science risk and deployment risk.
To make a strawman, it cannot just be a trillion dollars of venture capital driving climate tech. To illustrate this, just imagine if CRISPR was discovered in 2011 at a startup, rather than a university, and was never published! Even if there was a venture investor comfortable with the science risk of studying bacterial immune systems, the startup would have been alone in solving both genetic editing and delivery problems. It probably would have failed. Then the CRISPR IP would have been acquired by a large company, and locked away; the Innovator’s Dilemma limits the giants from trying anything too transformational. The billion-dollar gene editing ecosystem could have taken decades longer to emerge, if ever.
But what happened instead, fortunately, is that CRISPR discovery was published, the plasmids were made available on Addgene, and gene-editing boomed from 2012-2022. Billions of dollars of value was created and a whole ecosystem of specialists was born. CRISPR, in the sense of its original Cas9 instantiation, is now the kernel of a much broader ecosystem of modern biotech startups.
And, to make the other strawman, it cannot just be top-down programs that distribute all the capital, such as from governments: While roadmapping-based innovation is essential, the ecosystem growth must be organic and cannot be prescribed because the future of science cannot be predicted.
[8]
Let climate biotech refer to the field of research and practice of bioengineering toward a thriving biosphere. Climate biotech must grow organically like the gene editing ecosystem, but faster and across more verticals. And the climate biotech community must grow like the machine learning community has around benchmarks and challenges, but in atoms, not bits. Problems must be surfaced, platforms created to accelerate efforts toward problems, and pathways to scale must be developed: at the end of the day, it has to be practitioners leading the way.
So curing rare cancers in mice may be a focal point today but the economic gravity has to shift by 2030[9]. Imagine if it was actually a great time in biotech to be a whale inhaling heavy metals, or a pine tree fighting a beetle infestation, or a person in Ohio recovering from unknown chemicals spilled on him. What if you were that person? “Yes that was a dark spot,” you’d think, “but wow are we lucky to have the best people in the world working on our problems.”
Acknowledgements:
In no particular order, huge thanks to Alex Rosay at Cascade Biocatalysts, Cecilia Martinez-Gomez at UC Berkeley, Jenna Hua at Million Marker, Trevor Nicks at Caravel, David Ortega at Phase Biolabs, Henry Lee at Cultivarium, for their technical input and conversation.
Thanks to Niko McCarty for editing and, well, teaching me how to write.
Thank you to Miranda Stahn for technical and writing edits.
Special thanks to my brother (and Homeworld co-founder) Paul Reginato for being one of the deepest thinkers I know and for using his artist’s soul to always drive to beauty in both art and science. I appreciate his collaboration and support in using this blog space to prototype ideas.
1 According to this tweet summarizing the investment bank Evercore’s analysis of top genetic medicine programs, there are 50 gene editing programs working on Duchenne’s Muscular Dystrophy, which is ~$10-20B investment for a terrible disease that impacts 6 in 100,000 people. ↩
2 “Solarpunk” is not the perfect term, but it’ll do. Strictly speaking, solarpunk has it’s own aesthetic and culture, which I’m not explicitly endorsing. Please read “solarpunk” here as the stand-in for the yet-to-be-coined, punchy term for a positive future of both advanced technology and vibrant biosphere. ↩
3 Richard Hamming built the lexicon of “Most important problems” in his 1986 lecture, “You and Your Research.” He worked on his important problems within the framework of Bell Labs (funded by a government-granted monopoly) and speaks well to the ideals of always being oriented towards the most high impact, actionable challenges in any field. ↩
4 Self-replicating → infinitely scalable. ↩
5 David Ortega makes a fantastic point that biology is not just hard to engineer, it’s hard to even get a sense of how little we know. Consider the landmark minimal microbial genome paper in 2016: 149/473 genes were demonstrably essential to the microbe’s life yet had no known function! ↩
6 Not to mention graduate student careers. ↩
7 BASF, Bayer, Corteva and Syngenta are known as ‘The Big Four.’ ↩
8 Yes, this is a reference to Beginning of Infinity by David Deutsch. ↩
9 A good starting point would be ignoring those iBankers calling for medicine to look like subscription business, eg the infamous line “cures aren’t profitable”. ↩